Acoustics
Sound Waves and How They Move
Acoustics is the study of sound. In this section we're going to explore how sound waves travel from the vibrating vocal folds, through the vocal tract (throat), and out into the air.
Sound happens when air molecules get moved, and the movement continues in a wave (much more on that later). When the sound is continuous (like the human voice), it's because there's some vibration that moves, or disturbs, the air molecules. In the case of the human voice, it's the vocal folds that are vibrating. You actually know a lot about vibration, even if you've never thought about it.
Properties of Vibration
The most basic example of vibration is called simple harmonic motion. Simple harmonic motion is also known as sinusoidal motion. You may have heard of sine waves - they're created by sinusoidal motion.
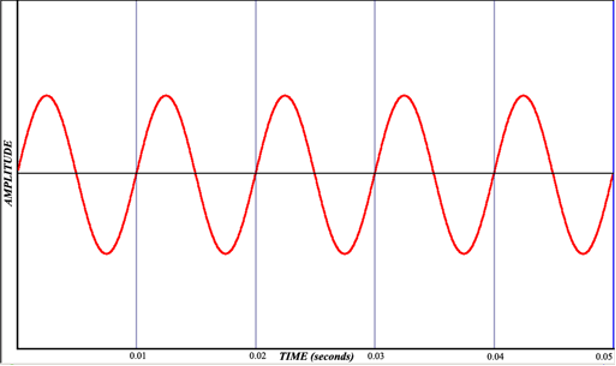
Figure 14: A sine wave - like the beginning of "The Outer Limits" for you boomers.
Think of a pendulum. It swings in one direction, slows and reverses its direction, swings back to its midline, and then swings in the opposite direction, the same distance as it moved in the original direction. Then it reverses direction, returns to midline, and begins the process again.
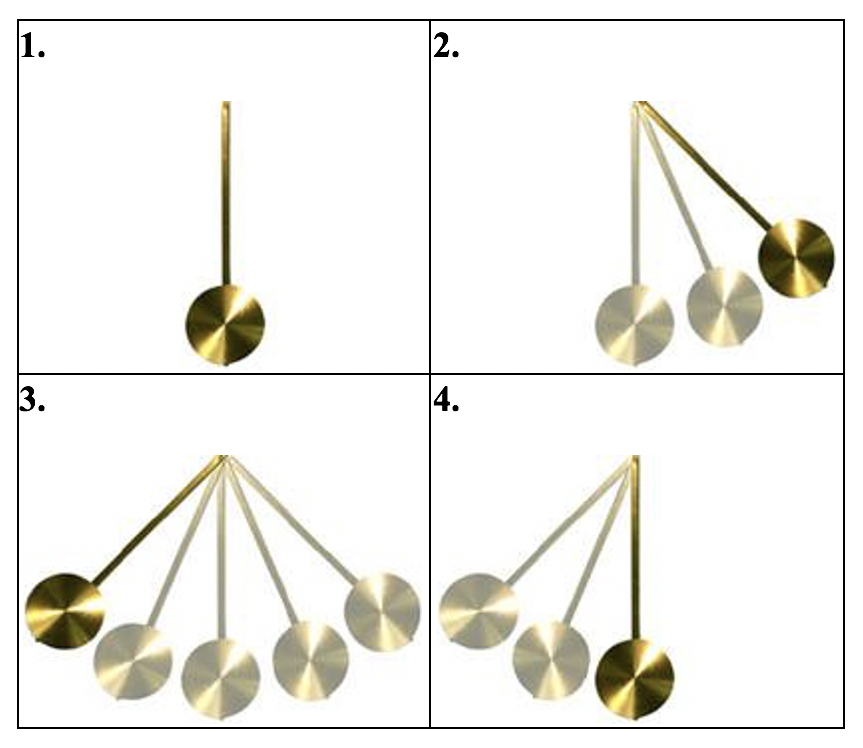
Figure 15: Movement of a Pendulum.
There are only three properties that can be manipulated with a swinging pendulum, and these factors help illustrate the properties of vibration.
Vibration Terms
Frequency is how many times per second the pendulum crosses its midline. Frequency measures the speed of vibration. Frequency is measured in Hertz (the abbreviation is Hz, but we always say "hertz"). In sound, frequency of vibration gives us the pitch of the sound. The faster the vibration, the higher the pitch that we perceive. So, we say that the perceptual correlate of frequency is pitch. Click here to see the equivalences of pitch and frequency. (Yes, vocal folds can vibrate faster than 1000 Hz, or 1000 vibrations per second!)
Amplitude is how far the pendulum swings away from its midline, in either direction. Amplitude measures the strength of vibration. Amplitude is measured in decibels (the abbreviation is dB, you can say decibels or dB, but not dB's - the abbreviation is already plural).
In sound, amplitude of vibration gives us the loudness of the sound. The greater the amplitude, the louder the sound we perceive. So we say that the perceptual correlate of amplitude is loudness.
BUT, there's not a direct correlation between amplitude and loudness the way there is for frequency and pitch. Acoustic scientists distinguish between the amplitude of vibration, the intensity (or sound pressure level -- we won't go into detail on this property in this section), of the sound wave, and the loudness of the sound perceived. Those are all measured differently, and don't correspond to each other in a one-to-one fashion. In the case of the voice, the amplitude of the vibration of vocal folds may determine the intensity of the sound wave, but many other factors influence our perception of the voice's loudness. Read on, and we'll discuss this topic more, but some of the discussion is beyond the scope of this website.
Phase refers to the moment in time the vibration starts its first excursion away from midline. Usually, this is in comparison to when other vibrations begin their first excursion away from midline. Phase doesn't actually measure any property of vibration. Rather, it's a property of vibration that exists at any moment in time.
Phase is measured in degrees away from midline, just like degrees of a circle. If one vibration starts it's excursion in one direction at exactly the same time another starts its excursion in the opposite direction, they are said to be 180 degrees out of phase.
In sound, phase doesn't have a perceptual correlate in the way frequency and amplitude do. If we could hear two simultaneous sine waves with the same frequency and amplitude but were out of phase with one another, we might only hear a slight buzziness to the sound. In the complex sounds we hear, we don't perceive the phase differences as a separate entity. Phase differences are very important in voice though, because different portions of the vocal folds may vibrate out of phase with each other, resulting in very complex vibration. More about that later…
For Your Information
So far we have been talking about listening to sine waves. In real life, though, we don't actually hear sine waves or simple harmonic motion. Rather, sounds that we hear are usually the result of complex vibration in which there are many simultaneous frequencies, amplitudes, and phase differences.
Confused? Pictures help!
A picture of vibration as it proceeds through time is called a waveform. A waveform shows properties of vibration.
Let's start with the most basic waveform, which is a picture of a sine wave (a.k.a. sinusoid, or simple harmonic motion).
Figure 16: Waveforms of sine waves: The horizontal axis represents time, and the vertical axis represents displacement from midline (which we'll compare to the excursion of the pendulum).
Movement of a Sound Wave
Sound Wave - A sound wave is a disturbance of air molecules, propagated through air.
For our discussion, assume the disturbance of air molecules will eventually disturb the tympanic membrane (ear drum) causing a vibration to go into the brain, where it is interpreted as sound.
The journey from the eardrum into the brain is very cool. Go look it up on the web!
Think of a slinky. If you hold one end still, and move the other in and out in a regular rhythm, each band of the slinky stays pretty much in place, just moving back and forth as much as you originally moved the end of the slinky back and forth. Each band of the slinky will run into the next band, and then move backward away from it. This will be repeated along the whole length of the slinky. But you'll see the bands as they bunch up, and the location of the bunched up section will continue to move to the other end of the slinky. It will look like a wave, traveling along the length of the slinky, over and over, as long as you continue to move the one end. (Now you want a slinky, don't you? Go buy one and play with it!)
The wave along the slinky is like a sound wave as it travels through air. Each air molecule stays in one place, bouncing back and forth, slamming into the molecule ahead of it, and then slamming into the molecule in back of it. Molecules moving in a forward direction will get bunched up, or compressed, and then more spaced apart, or rarefacted, as they bounce backward away from each other. The compressions (bunched up areas) keep moving along, eventually dying out due to the friction of the air, or ending up on someone's ear drum.
In case this sounded too easy……The molecules moving forward create compressions, and the molecules bouncing backward create rarefactions, BUT, when the backward moving molecules bump into forward moving molecules just behind them, that might create another compression, depending on how soon the next wave starts and how far the molecules are moving (which depends on the amplitude of the original disturbance). And, if the air molecules run into a surface that deflects them, they can start a whole series of compressions and rarefactions moving away from that surface. And in case that wasn't complex enough, air molecules, unlike bands in a slinky, can move in any direction. This means that, as all the air molecules surrounding the disturbance are moved, the sound wave travels outward in ALL directions; those compressions and rarefactions can run into anything, anywhere, and be deflected back from who-knows-where.
That all makes sense when you consider the sound you make when you yell across a large, open space. But you probably didn't think about the sound wave as it leaves your vibrating vocal folds and travels through your vocal tract. That sound wave travels in a very complex pattern of compressions and rarefactions, and when the pressure hits structures like the roof of your mouth and your teeth, it's deflected back, and can exert pressure back onto your vocal folds, affecting the way they vibrate. That ends up being pretty important.
AND - all of this happens really fast. The disturbance of air molecules travels at the speed of sound: 350 meters per second, or 35,000 centimeters per second. Since the average vocal tract is about 17 centimeters from vocal folds to the lips, the disturbance whips through your throat REALLY fast.
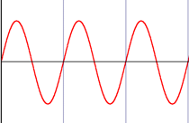
Figure 17: Remember the waveform - the picture of a sound wave?
Think of the waveform as depicting compressions and rarefactions. The positive portion of the waveform is the compression (where there's positive pressure) and the negative portion is the rarefaction (where there's negative pressure). The waveform shows how the compressions and rarefactions move through time, but remember that in any one place, the air molecules are alternately compressed and rarefacted.
Wavelength - One more concept to think about before we get to the really cool stuff: wavelength is how far a compression travels before the next compression starts. The compressions travel at the speed of sound, so the wavelength depends upon the frequency of vibration, that is, how quickly the next compression starts.
More on acoustics coming soon!
For Appointments:
Call: 612-626-5900
Address
M Health Fairview Clinics and Surgery Center - Minneapolis
909 Fulton St. SE
Minneapolis, MN 55455
Interesting Fact
Oh, by the way, if a tree falls in the forest, there's a sound wave that travels through the air, whether there's an ear drum to hear it or not.